BNL main QIS activities and links:
BNL Quantum Information Science and Technology portal
BNL C2QA quantum center Jim Misewich @ P5 2023
CSI Quantum Computing Group
- Long Island Quantum Distribution Network, LIQuIDNet
Links to other BNL QIS projects and results, which have overlap with the quantum astrometry project.
Free-space quantum link between BNL and SBU
need an IO confluence account to see the pages
Quantum Internet
- Building a Network for Long-Distance Quantum Communication BNL newsroom August 2019
- An elementary 158 km long quantum network connecting room temperature quantum memories Dounan Du, Paul Stankus, Olli-Pentti Saira, Mael Flament, Steven Sagona-Stophel, Mehdi Namazi, Eden Figueroa https://arxiv.org/abs/2101.12742
- Spatial and temporal characterization of polarization entanglement Nomerotski, A.; Katramatos, D.; Stankus, P.; Svihra, P.; Cui, G.; Gera, S.; Flament, M.; Figueroa, E., International Journal of Quantum Information 2020, 18, 1941027; doi:10.1142/s0219749919410272. .pdf
We describe the full temporal and spatial characterization of polarization-entangled photons produced by Spontaneous Parametric Down Conversions using an intensified high-speed optical camera, Tpx3Cam. This novel technique allows for precise determination of Bell inequality parameters and for new characterization methods for the spatial distribution of entangled quantum information. We also discuss a technique to synchronize multiple cameras separated by vast distances, which will be required for a distributed quantum network.
- Fast camera spatial characterization of photonic polarization entanglement, Ianzano, C.; Svihra, P.; Flament, M.; Hardy, A.; Cui, G.; Nomerotski, A.; Figueroa, E., Fast camera spatial characterization of photonic polarization entanglement, Scientific Reports 2020, 10; doi:10.1038/s41598-020-62020-z.
Scalable technologies to characterize the performance of quantum devices are crucial to creating large quantum networks and quantum processing units. Chief among the resources of quantum information processing is entanglement. Here we describe the full temporal and spatial characterization of polarization-entangled photons produced by Spontaneous Parametric Down Conversions using an intensified high-speed optical camera, Tpx3Cam. This novel technique allows for precise determination of Bell inequality parameters with minimal technical overhead, and for new characterization methods for the spatial distribution of entangled quantum information. The fast-optical camera could lead to multiple applications in Quantum Information Science, opening new perspectives for the scalability of quantum experiments.
- Quantum Networks For Open Science, T. Ndousse-Fetter et al., arXiv:1910.11658 [quant-ph], Oct 2019.
The United States Department of Energy convened the Quantum Networks for Open Science (QNOS) Workshop in September 2018. The workshop was primarily focused on quantum networks optimized for scientific applications with the expectation that the resulting quantum networks could be extended to lay the groundwork for a generalized network that will evolve into a quantum internet.
Ion trapping in storage rings for quantum computers
Cold ion beam in a storage ring as a platform for large-scale quantum computers and simulators: challenges and directions for research and development, Timur Shaftan, Boris B. Blinov, https://arxiv.org/abs/2101.04247
The purpose of this paper is to evaluate the possibility of constructing a large-scale storage-ring-type ion-trap system capable of storing, cooling, and controlling a large number of ions as a platform for scalable quantum computing (QC) and quantum simulations (QS). In such a trap, the ions form a crystalline beam moving along a circular path with a constant velocity determined by the frequency and intensity of the cooling lasers. In this paper we consider a large leap forward in terms of the number of qubits, from fewer than 100 available in state-of-the-art linear ion-trap devices today to an order of 105 crystallized ions in the storage-ring setup. This new trap design unifies two different concepts: the storage rings of charged particles and the linear ion traps used for QC and mass spectrometry. In this paper we use the language of particle accelerators to discuss the ion state and dynamics. We outline the differences between the above concepts, analyze challenges of the large ring with a revolving beam of ions, and propose goals for the research and development required to enable future quantum computers with 1000 times more qubits than available today. The challenge of creating such a large-scale quantum system while maintaining the necessary coherence of the qubits and the high fidelity of quantum logic operations is significant. Performing analog quantum simulations may be an achievable initial goal for such a device. Quantum simulations of complex quantum systems will move forward both the fundamental science and the applied research. Nuclear and particle physics, many-body quantum systems, lattice gauge theories, and nuclear structure calculations are just a few examples in which a large-scale quantum simulation system would be a very powerful tool to move forward our understanding of nature.
- Towards storage rings as quantum computers, K. A. Brown and T. Roser, Phys. Rev. Accel. Beams 23, 054701 (2020)
We explore the possible use of particle beam storage rings as quantum computers. More precisely, we consider creating an ion trap system, in which the same computational basis states can be defined as in a modern ion trap system, but in which the ions have a constant velocity and are rotating in a circular trap. The basic structures that we explore are classical and ultracold crystalline beams. What we propose is a novel method that uses the ion trap quantum computer concept, but puts the ions into a rotating frame of reference. The benefits of this approach are discussed.
Ion crystals in RF (Paul) traps
Micromotion-Synchronized Pulsed Doppler Cooling of Trapped Ions; Alexander Kato, Andrei Nomerotski, and Boris B. Blinov
We propose and demonstrate a new method for Doppler cooling trapped-ion crystals where the distribution of micromotion amplitudes may be large and uneven. The technique uses pulses of Doppler cooling light synchronized with the trap RF that selectively target ions when their velocity is near a node, leading to more uniform cooling across a crystal by a single tone of cooling light. We lay out a theoretical framework that describes where this technique is practical, and provide a simple experimental demonstration.
- Two-tone Doppler cooling of radial two-dimensional crystals in a radiofrequency ion trap, Alexander Kato, Apurva Goel, Raymond Lee, Zeyu Ye, Samip Karki, Jian Jun Liu, Andrei Nomerotski, Boris B. Blinov; Phys. Rev. A 105, 023101
We study the Doppler-cooling of radial two-dimensional (2D) Coulomb crystals of trapped barium ions in a radiofrequency trap. Ions in radial 2D crystals experience micromotion of an amplitude that increases linearly with the distance from the trap center, leading to a position-dependent frequency modulation of laser light in each ion's rest frame. We use two tones of Doppler-cooling laser light separated by approximately 100~MHz to efficiently cool distinct regions in the crystals with differing amplitudes of micromotion. This technique allows us to trap and cool more than 50 ions in a radial two-dimensional crystal. We also individually characterize the micromotion of all ions within the crystals, and use this information to locate the center of the trap and to determine the Matthieu parameters qx and qy.
- Direct Observation of Ion Micromotion in a Linear Paul Trap, Liudmila Zhukas, Maverick Millican, Peter Svihra, Andrei Nomerotski, Boris Blinov, https://arxiv.org/abs/2010.00159, Phys. Rev. A 103, 023105 (2021).
In this paper, the first direct observation of micromotion for multiple ions in a laser-cooled trapped ion crystal is discussed along with a novel measurement technique for micromotion amplitude. Micromotion is directly observed using a time-resolving, single-photon sensitive camera that provides both fluorescence and position data for each ion on the nanosecond time scale. Micromotion amplitude and phase for each ion in the crystal is measured which allows this method to be sensitive to tilts and shifts of the ion chain from the null of the radiofrequency quadrupole potential in the linear trap. Spatial resolution makes this micromotion detection technique suitable for complex ion configurations, including two-dimensional geometries. It does not require any additional equipment or laser beams, and the modulation of the cooling lasers or trap voltages is not necessary for detection, as it is in other methods.
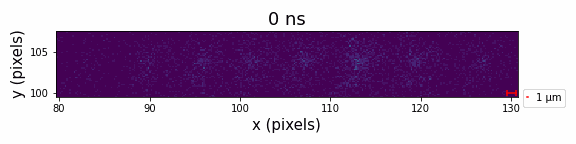
High-Fidelity Simultaneous Detection of Trapped Ion Qubit Register, Liudmila A. Zhukas, Peter Svihra, Andrei Nomerotski, Boris B. Blinov, https://arxiv.org/abs/2006.12801, June 2020; Phys. Rev. A 103, 062614
Qubit state detection is an important part of a quantum computation. As number of qubits in a quantum register increases, it is necessary to maintain high fidelity detection to accurately measure the multi-qubit state. Here we present experimental demonstration of high-fidelity detection of a multi-qubit trapped ion register with single qubit detection fidelity of 0.99995(+3/-8) and 4-qubit state detection fidelity of 0.9991(+5/-15) using a novel single-photon-sensitive camera with fast data collection, excellent temporal and spatial resolution, and low instrumental crosstalk.
Quantum Optics with single photon imaging and time stamping
Baptiste Courme, Chloé Vernière, Peter Svihra, Sylvain Gigan, Andrei Nomerotski, Hugo Defienne
High-dimensional entanglement is a promising resource for quantum technologies. Being able to certify it for any quantum state is essential. However, to date, experimental entanglement certification methods are imperfect and leave some loopholes open. Using a single-photon sensitive time-stamping camera, we quantify high-dimensional spatial entanglement by collecting all output modes and without background subtraction, two critical steps on the route towards assumptions-free entanglement certification. We show position-momentum Einstein-Podolsky-Rosen (EPR) correlations and quantify the entanglement of formation of our source to be larger than 2.8 along both transverse spatial axes, indicating a dimension higher than 14. Our work overcomes important challenges in photonic entanglement quantification and paves the way towards the development of practical quantum information processing protocols based on high-dimensional entanglement.
High Speed Imaging of Spectral-Temporal Correlations in Hong-Ou-Mandel Interference, Yingwen Zhang, Duncan England, Andrei Nomerotski, Benjamin Sussman
In this work we demonstrate spectral-temporal correlation measurements of the Hong-Ou-Mandel (HOM) interference effect with the use of a 3D (2 spatial + 1 time dimension) camera based spectrometer. This setup allows us to take, within seconds, spectral temporal correlation measurements on entangled photon sources with sub-nanometer spectral resolution and nanosecond timing resolution. Through post processing, we can observe the HOM behaviour for any number of spectral filters of any shape and width at any wavelength over the observable spectral range. Our setup also offers great versatility in that it is capable of operating at a wide spectral range from the visible to the near infrared and does not require a pulsed pump laser for timing purposes. This work offers the ability to gain large amounts of spectral and temporal information from a HOM interferometer quickly and efficiently and will be a very useful tool for many quantum technology applications and fundamental quantum optics research.
Ghost imaging with x-rays